You may be worried about vegetables and fruits being spoiled unknowingly or you may be confused when the buds at your garden may bloom. But it seems as your worries are now over. Thanks to a team of researchers at the Massachusetts Institute of Technology (MIT) who stepped closer to solve this problem by using carbon nanotubes.
The researchers describing their work was published in the journal ACS Central Science.
It is estimated that upward of 50% of a farm’s production value may be lost as a result of various issues along the supply chain. Ethylene monitoring could reduce losses if changes in plant health are detected at an early stage and preventative actions are taken.
Though we know we have to take into account the ethylene present in plants, we are far from an effective method and technology that can track them.
Timothy Swager, a materials chemist at the Massachusetts Institute of Technology (MIT) said, "There is a persistent need for better food management and reduction of food waste." Swager added, "People who transport fruit around would like to know how it’s doing during transit, and whether they need to take measures to keep ethylene down while they’re transporting it."
Ethylene is something in plants that trigger germination, flowering, ripening and rotting in seeds, flowers, fruit, and vegetables, and it’s also released during these processes. Ethylene allows plants to communicate and synchronize so to forecast which products to sell first, and how to spot rot before it spreads it’s something the food and flower industries would love to detect early. Swager said, "There still is not a good commercial sensor for ethylene. To manage any kind of produce that’s stored long-term, like apples or potatoes, people would like to be able to measure its ethylene to determine if it’s in a stasis mode or if it’s ripening."
Timothy Swager, Darryl Fong, and colleagues at MIT and the Nanotechnology National Laboratory for Agriculture in Brazil used carbon nanotubes (CNTs) in combination with a highly selective catalyst and successfully designed a sensor that can detect ethylene at concentrations of as little as 15 parts per billion.
Previous approaches to ethylene sensing were typically based on using photoacoustic spectroscopy (which detects sounds produced in response to light) or gas chromatography (which separates chemicals based on their different retention times in solvents). Also, we have techniques like turn-on fluorescence with luminescent polymers or olefin metathesis catalyst/fluorescent dye hybrid molecules, chemiresistors, graphene-based field-effect transistors, electrochemical oxidation, and amperometry to detect ethylene.
But for each of these techniques there exist major limitations. Each technique includes complicated sensor preparation works or low sensitivity or humidity intolerance and/or sensitivity to oxygen. So, neither technique is easy or simple enough for a grocer or florist to incorporate it into their work.
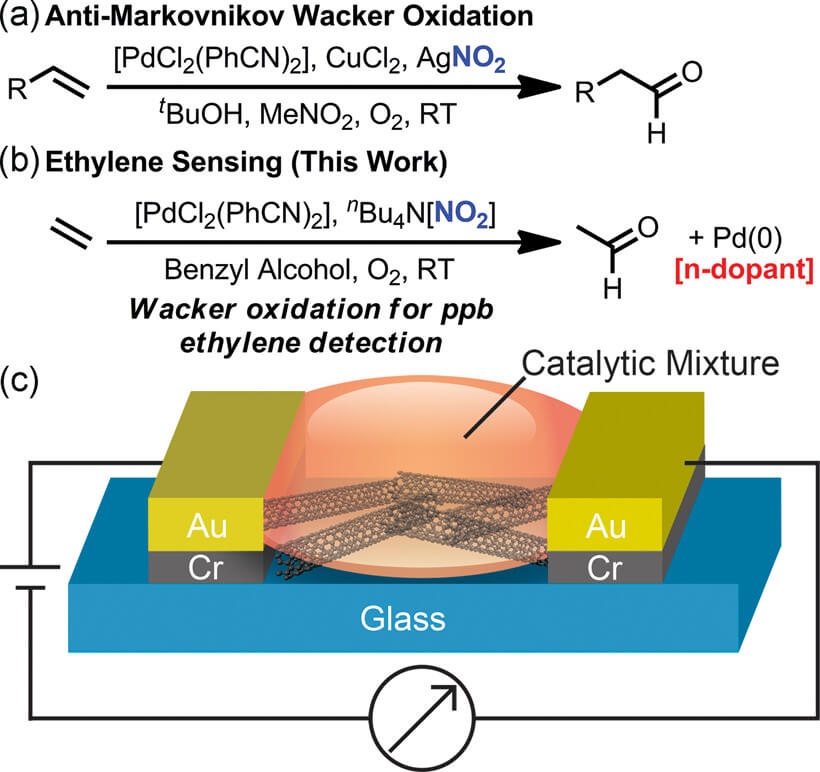
To trace ethylene under diverse ambient conditions Swager and colleagues designed a highly selective ethylene chemiresistive sensor that can detect parts per billion (ppb) levels of ethylene. The sensor designed by them is prepared from single-walled carbon nanotubes (SWCNTs) for which its conductance strictly depends on carrier densities. Especially, Fong and Swager worked with CNTs of p-type semiconductors so n-type dopants - anything that donates electrons to the CNT - will diminish their conductivity. The curved graphene surface of CNT’s also makes their electronic properties incredibly sensitive to dopants in their environment.
Swager said, "There’s a graveyard of CNT sensors with low specificity." He added "You need a way of boosting the signal above the noise," because the CNTs are so sensitive to everything in the environment, he explains, all you detect is white noise.
In the course of searching for specificity and sensitivity they require, researchers stuck at the well-known process called the Wacker oxidation process in which ethylene - a hydrocarbon containing two double-bonded carbon atoms - oxidizes into acetaldehyde.
First, they tried to mimic the plants themselves, which use copper ions to promote ethylene activity. But as plants have a natural ability to keep copper in its Cu(I) oxidation state - which has one fewer electron than the material would have as a neutral atom - this process proved to a more convoluted process. Therefore, in a synthetic device, copper tends to exist in the Cu(II) oxidation state, and preventing Cu(I) from oxidizing to Cu(II) proved too difficult for this approach to have commercial potential. However, in the Wacker reaction, ethylene oxidation is catalyzed by palladium in the Pd(II) state, and, from the study, this is more stable than Cu(I). Using this process, they found that the resulting ethylene reaction surrenders electrons that reduce Pd(II) to the n-dopant Pd(0), which the highly sensitive CNTs should announce with a dip in conductivity from the excess electrons.
They found that the ethylene profiles taken from the experiments coincides with the observed blooming times for the flower populations and are in agreement with the known low ethylene sensitivity of lisianthus flowers and high ethylene sensitivity of carnations. Furthermore, the team also tested whether a commercial flower nutrient package provided by the carnation supplier has an impact on the ethylene emission profile. For this the carnations were (i) left on the table without water, (ii) treated with water only, or (iii) treated with nutrient water. They observed no change in ethylene emission when the carnations are not treated with water. Meanwhile, treatment with water results in peak ethylene emission about 4 h earlier than treatment with nutrient water, which suggests that the nutrient packages do not strongly influence the ethylene emission profile.