By aligning the atomic lattice of a graphene layer to that of an insulating boron nitride sheet, a team of researchers from the University of Manchester has discovered and characterised a new family of quasiparticles - named Brown-Zak fermions.
The work describes how electrons behave in an ultra-high-quality superlattice of graphene with a revised framework for the fractal features of the Hofstadter’s butterfly. Fundamental improvements in graphene device fabrication and measurement techniques in the past decade have made this work possible.
It is well-understood and observed that in quantizing magnetic fields, graphene superlattices exhibit a complex fractal spectrum often referred to as the Hofstadter butterfly.
Hofstadter butterfly model gives the spectral properties of non-interacting two-dimensional electrons in a magnetic field in a lattice and plays a crucial role in the theory of the integer quantum Hall effect and the theory of topological quantum numbers.
It was Van der Waals assembly idea that offers a possibility to create materials by stacking atomically-thin layers of different crystals and one of the simplest and most studied Van der Waals heterostructures is graphene encapsulated between two hexagonal boron nitride (hBN) crystals where the encapsulation protects graphene from extrinsic disorder and allows ultra-high electronic quality and micrometre-scale ballistic transport often limited only by edge scattering.
Here the team assembled a trilayer graphene-on-hBN (thickness being between 20 and 70 nm) superlattices using the standard dry transfer procedures and polydimethylsiloxane (PDMS) stamps coated with a polypropylene carbonate (PPC) layer.
This achievement is a successive storey fundamentally laying on the advancement of graphene-boron nitride superlattices, which allowed the observation of a fractal pattern but this time they notice something abnormal behaviour of the particles when the magnetic field was applied.
Piranavan Kumaravadivel, a research member, said, “Such behaviour is radically different from textbook physics.
Julien Barrier, another research member explained, “It is well known that in zero magnetic field electrons move in straight trajectories and if you apply a magnetic field, they start to bend and move in circles. In a graphene layer which has been aligned with the boron nitride, electrons also start to bend - but if you set the magnetic field at specific values, the electrons move in straight line trajectories again, as if there is no magnetic field anymore!”
Authors proposed Brown-Zak fermions to be the family of quasiparticles existing in superlattices under high magnetic field. Heretofore, the behaviour of collective electrons in graphene superlattice used to be described in terms of Dirac fermion, however, did not account for some other important features like the additional degeneracy of the states, nor did it match the finite mass of the quasiparticle in this state.
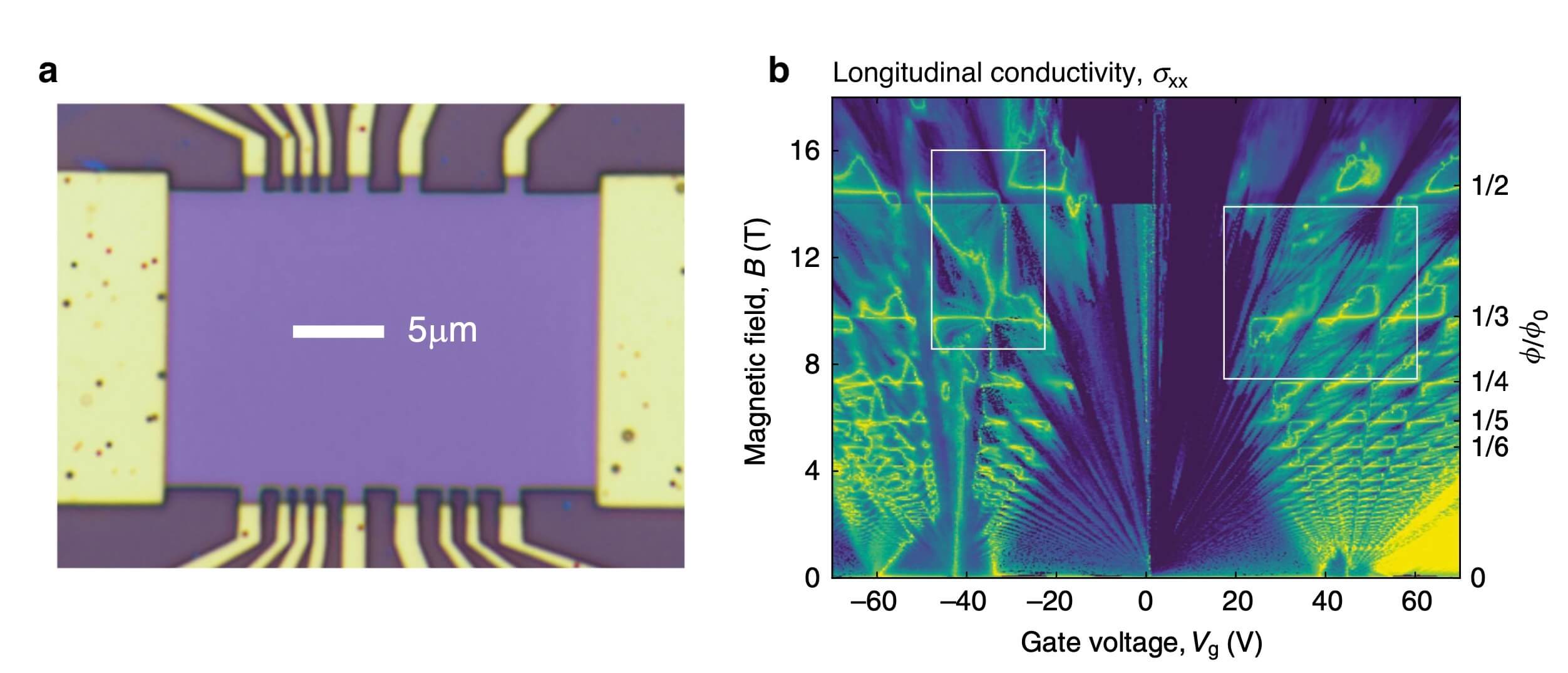
Julien Barrier describes, “The concept of quasiparticles is arguably one of the most important in condensed matter physics and quantum many-body systems. It was introduced by the theoretical physicist Lev Landau in the 1940s to depict collective effects as a ‘one particle excitation. They are used in a number of complex systems to account for many-body effects.”
Brown-Zak fermions to be the family of quasiparticles was characterised by a new quantum number that can directly be measured. Interestingly, working at lower temperatures allowed them to lift the degeneracy with exchange interactions at ultra-low temperatures.
Kumaravadivel explained, “Under the presence of a magnetic field, electrons in graphene start rotating with quantised orbits. For Brown-Zak fermions, we managed to restore a straight trajectory of tens of micrometres under high magnetic fields up to 16T (500,000 times earth’s magnetic field). Under specific conditions, the ballistic quasiparticles feel no effective magnetic field.”
The new form of material has a high level of purity hence showed very high mobility.
High level of purity of the new material allowed the team to achieve mobilities of several millions of cm²/Vs. This means particles would travel straight across the entire device without scattering.
Importantly, this was not only the case for classical Dirac fermions in graphene but also realised for the Brown-Zak fermions reported in the work.
Materials with high mobilities have long been the Holy Grail when fabricating 2D systems such as graphene because such materials would present additional properties (integer and fractional quantum hall effects), and potentially allow the creation of ultra-high frequency transistors, the components at the heart of a computer processor.
The high mobility means that a transistor made from such a device could operate at higher frequencies, allowing a processor made out of this material to perform more calculations per unit of time, resulting in a faster computer.
Julien Barrier added, “The findings are important, of course for fundamental studies in electron transport, but we believe that understanding quasiparticles in novel superlattice devices under high magnetic fields can lead to the development of new electronic devices.”
Research work was published in the journal Nature Communications on 13 November 2020.